Striking elegance of molecular landscapes
Molecular biologists study an invisible world. A perplexing choas of molecules that together give rise to the complexity of life. Is it possible to illustrate the intricacies of our molecular organization?
Most people with an interest in the natural sciences know that a living being is made up of billions of cells in which complex processes are constantly taking place. In high school, during biology class, we are imprinted with terms like "powerhouse of the cell" (mitochondrion), "protein factory" (ribosome), "control center" (nucleus); all metaphors to make the baffling complexity of the cell somewhat tangible. Illustrations in biology textbooks are also almost always simplified to the most basic essence. Sure, it makes the matter comprehendible, but it also minimizes our understanding of how the millions of molecules in a living system interact.
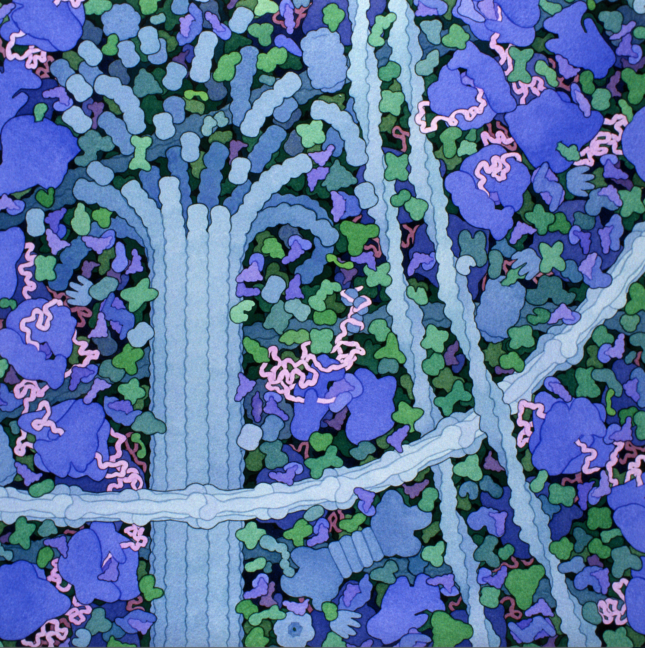
Even after years of academic study, it is not clear how this jungle of proteins and molecular networks can be clearly depicted. David Goodsell, an American biochemist and structural biologist, tries to help us in this effort. Since his college days, he has dedicated himself to the question: if I could dive into a living cell, what would I actually see? Using his knowledge of the molecular world as well as his artistic talent, he creates hyper-realistic paintings that faithfully depict the lush, teeming contents of cells.

Goodsell considers it extremely important that his drawings accurately reflect reality. Much of his time is spent gathering information, studying the structure of molecules, their interactions with each other, and their place within the cell. An important source of information on which he bases his work is the Protein Data Bank, a database containing tens of thousands of biological molecules. The spatial shape of a molecule can be determined using various techniques, such as X-ray crystallography. This is a research technique that, based on the scattering of X-rays, can precisely unravel the atomic structure of a material, such as a protein or nucleic acid. Goodsell sketches all the molecules that will appear in his drawings, so that he can get an idea of their respective sizes and shapes.
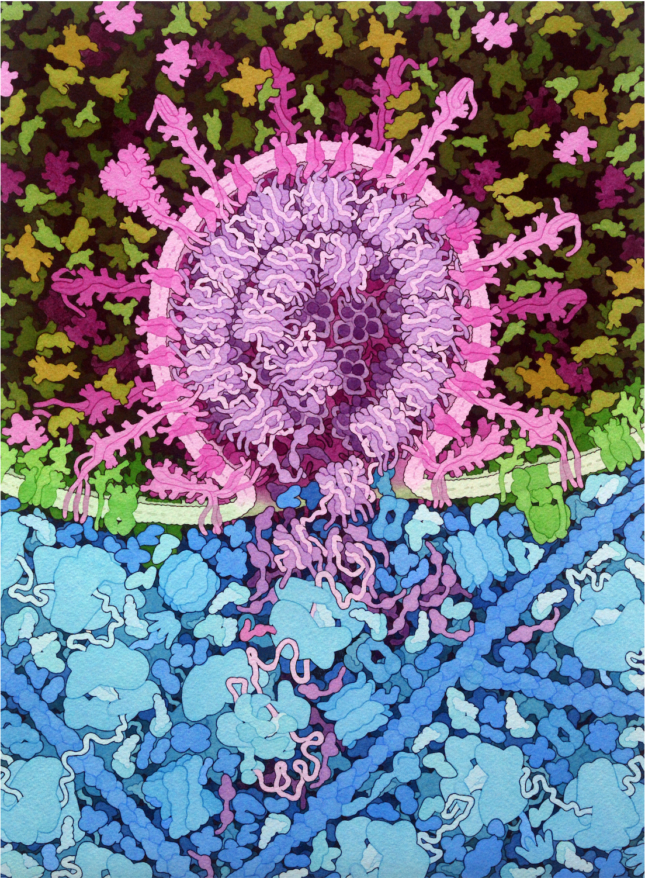
For a long time, it was virtually impossible for humans to view individual molecules under a microscope. Light waves are simply too large; they wave around the atoms. With the invention of the electron microscope around 1940, this diffraction limit could be passed for the first time. However, electron microscopes have many drawbacks. The specimen must be killed and preserved, then placed in a vacuum chamber. As a consequence, little remains of the living, dynamic cellular environment. How all the molecules are related in the context of the living cell is still impossible to visualize with modern techniques. Goodsell's drawings give us a faithful impression.

Goodsell's drawings have found their way into textbooks, research publications and covers of science journals like Nature. They show that the beauty of molecular biology comes down not only to the theory, but also, perhaps more importantly, the elegance and sophistication of the objects themselves.
Want to learn and see more of this scientist? Every month – for over twenty years I might add – he posts a short introductory article about a certain macromolecule on the Protein Data Bank, the Molecule of the Month.

0 Comments
Add a comment